When spacecraft return to Earth, they don’t need to shed all their velocity by firing retro-rockets. Instead, they use the atmosphere as a brake to slow down for a soft landing. Every planet in the solar system except Mercury has enough of an atmosphere to allow aerobraking maneuvers, and could allow high-speed exploration missions. A new paper looks at the different worlds and how a spacecraft must fly to take advantage of this “free lunch” to slow down at the destination.
Aerocapture is an orbital transfer maneuver in which a spacecraft makes a single pass through a planetary atmosphere to decelerate and achieve orbit insertion. On the other hand, aerobraking uses a propulsive burn plus repeated dips into the atmosphere—i.e., atmospheric drag—to gradually slow the spacecraft and reduce the size of the orbit to achieve orbit insertion.
The new paper posted to the arXiv preprint server, by Athul Pradeepkumar Girija from the School of Aeronautics and Astronautics at Purdue University, notes that one of the significant risks associated with aerocapture is the uncertainty in the atmospheric density. While aerobraking takes place in the tenuous upper atmosphere of a planetary body where the density uncertainties are much larger, aerocapture occurs in the deeper atmosphere where the density uncertainties are smaller.
For example, the atmospheric density that the Mars Reconnaissance Orbiter MRO actually experienced when aerobraking for its orbital insertion in 2006 was much different than what was predicted by a NASA model called GRAM (Global Reference Atmospheric Model) for Mars.
“At some points in the atmosphere, we saw a difference in the atmospheric density by a factor of 1.3, which means it was 30% higher than the model,” said Han You, Navigation Team Chief for MRO, in an article on Universe Today in 2006. “That’s quite a bit, but around the south pole we saw an even larger scale factor of up to 4.5, so that means it was 350% off of the Mars GRAM model.”
For either aerobraking or aerocapture, the atmospheric density on Mars and other planets can vary widely from day to day, and even orbit to orbit.
“If the vehicle enters too shallow or encounters an atmosphere which is less dense than the expected minimum, spacecraft may exit the atmosphere without getting captured,” Girija wrote in his new paper. “If the vehicle enters too steep, or the density is much higher than expected, the vehicle may bleed too much speed and fail to exit the atmosphere.”
Both scenarios would lead to complete loss of mission. Therefore, adequate margins must be provided for the guidance system against these atmospheric uncertainties, in addition to delivery error and aerodynamic uncertainties.
To perform aerocapture, there are two kinds of aerodynamic control methods to control the rate of energy depletion as the vehicle flies through the atmosphere: lift modulation and drag modulation.
“Lift modulation involves a ‘lifting’ aeroshell such as Apollo or Mars Science Laboratory aeroshell, which has a lift-to-drag (L/D) ratio in the range of 0.24—0.36,” explained Girija in an email to Universe Today. “Control is achieved by ‘banking’ the vehicle to fly deeper into the denser atmosphere, or higher into the thinner atmosphere. This control method requires the use of high-rate reaction control thrusters and is routinely used at Earth and Mars, and has extensive heritage in Apollo and MSL (Mars Science Laboratory) missions.”
Lift modulation offers continuous control through the atmospheric flight while the reaction control guidance tries to achieve the desired “exit state conditions.”
Drag modulation, on the other hand, is a simpler control technique in which the control is achieved by continuous or discrete (occasional) modulation of the drag area using a deployable device.
“Drag modulation vehicles have L/D = 0, i.e. no lifting capability,” Girija said. “The most common variant is a ‘discrete event modulation’ where a deployed drag skirt is jettisoned during the flight, with the jettison time being the only control variable.”
By jettisoning the drag skirt at the correct time, Girija explained, it is possible to target a reasonably close exit state condition to what is ideal.
“Drag modulation has been proposed as a ‘cheaper’ alternative to lift modulation,” Girija said, “by avoiding the use of RCS thrusters and is particularly attractive for small missions. Drag modulation has no flight heritage, though some of the basic technologies have been demonstrated in flight experiments such as the Adaptable Deployable Entry and Placement Technology (ADEPT),” which had a successful test flight in September of 2018.
Another thing to consider is the entry corridor, which is the region of the atmosphere a spacecraft enters to reach its desired destination. The Theoretical Corridor Width (TCW) quantifies the width of the corridor, and must be large enough to accommodate a safe landing, accounting for atmospheric uncertainties, and also provide sufficient safety margin for mission success even in limiting scenarios, such as combination of shallow entry and thin atmosphere.
As a general rule of thumb, Girija said, lift modulation provides nearly twice the available entry corridor width as drag modulation, and can thus accommodate larger atmospheric uncertainties. The main difference is that while drag modulation offers somewhat less control, it is more affordable for small missions (less than $50 million) while lifting aeroshells typically cost several hundreds of millions of dollars.
Girija says that even though the atmospheres of Venus, Mars, and Titan are well-characterized for engineering purposes, there can be standard density variations of up to 50%, plus or minus. With no in-situ data, the atmospheres of Uranus and Neptune are not as well characterized, but the GRAM model for them provides a standard deviation variation of plus or minus 30%. An understanding of the expected uncertainties in the density profile is of great importance to assess the risk it poses to a future mission.
The GRAM model uses available in-situ and remote sensing measurements and provides an “engineering model for the planetary atmospheres,” Girija said. “For planets such as Mars and Venus, there is a lot of data (both in situ and remote sensing) and the models are considered quite reliable for preliminary engineering design. For Uranus and Neptune, there is no in-situ data available and the models are based solely on remote sensing observations during the Voyager flyby.”
But there is great diversity in the physical structure and chemical composition of the atmospheric layers of the planets in our solar system, from the “hot thick Venusian CO2 atmosphere to the cold icy H2-He atmospheres of Uranus and Neptune,” writes Girija, adding that measurements such as the noble gas abundances and isotopic ratios in these atmospheres are not only critical any aerobraking operations, but also to our understanding of the origin, formation, and evolution of the solar system.
For Venus’ thick atmosphere, aerocapture using its atmosphere has been shown to be feasible using both lift and drag modulation. However, the large heating rates at Venus make lift modulation not as desirable. Girija says that drag modulation with its lower heating rate particularly makes it attractive for small satellite orbit insertion.
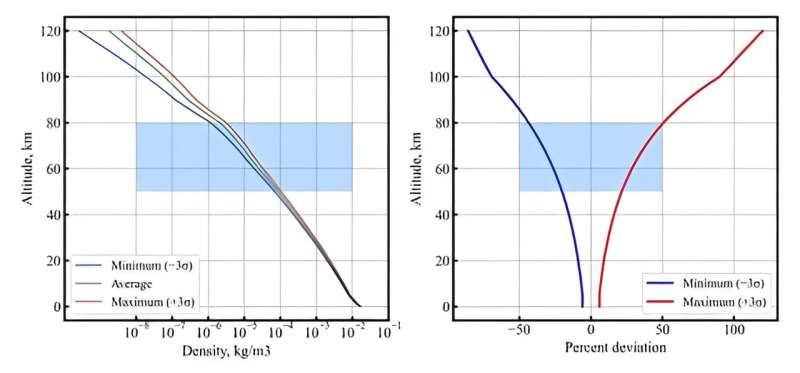
Mars has a relatively thin atmosphere compared to the Earth, but several missions have successfully used aerocapture for both orbit insertion and landing. Because of the numerous mission to Mars, the Martian atmosphere is well understood, but also has relatively large seasonal variations compared to Venus, and associated uncertainties particularly in the thinner upper atmosphere.
However, compared to Venus, the low gravity and the extended atmosphere provide larger TCW at Mars (by a factor of 2), and Girija says the larger atmospheric uncertainties can easily be accommodated. The “sweet spot” deceleration at Mars is a band of atmosphere between 50–80 km in altitude, where most of the deceleration occurs for aerocapture at Mars. For any mission to the Red Planet, the entry proposal needs to have adequate margin for two limiting scenarios: shallow entry and thin atmosphere, and thick atmosphere and steep entry.
Saturn’s largest moon Titan is the only moon in our solar system with an atmosphere. With surface liquids and its Earth-like terrain, Titan is an enticing world to study with a future mission. Girija says that Titan’s low gravity and extended thick atmosphere make it the ideal destination for aerocapture, and these conditions provide the largest corridor width of any destination in our solar system. Since its small size makes it particularly difficult to insert orbiters using conventional propulsion, aerocapture is a promising alternative for future missions that might perform global mapping of Titan’s surface and its lakes and seas. We do have the in-situ data from the Huygens lander, so Girija says that Titan’s density profile is fairly well constrained, with a few exceptions.
“The uncertainty in the density profile increases with altitude, reaches a maximum of about 40% near 100 km above the surface and then decreases,” Girija writes. “It is not clear this is an artifact of the assumptions used in the model, or indeed a real effect.”
The altitude band of 300–450 km is where most of the deceleration occurs for aerocapture at Titan, with a density variation of about 30%, which is comparable to Venus. Girija says that although Venus’ and Titan’s atmosphere are quite different in terms of their temperature (737K vs. 94K) and chemistry (CO2 vs. N2), they share several physical similarities, such as both being relatively thick, super-rotating atmospheres with the planetary body rotating slowly and significant greenhouse warming in the lower troposphere.
The ice giants Uranus and Neptune are the last class of planets yet to be explored using orbiter spacecraft. Even though their distance from Earth presents significant mission design challenges, the 2023–2032 Planetary Science Decadal Survey has identified a Uranus Orbiter and Probe as the top priority for a flagship mission in the next decade.
While Uranus and Neptune are both equally compelling scientifically, Girija says that Uranus is less demanding from a mission design perspective with propulsive insertion. “Aerocapture has been shown to be strongly enhancing to enabling technology for ice giant missions,” he writes. “With aerocapture, both Uranus and Neptune would be equally accessible. Recent studies have shown that aerocapture enables significantly shorter flight times to Uranus than possible with propulsive insertion, especially with new high energy launch vehicles.”
For both Uranus and Neptune, the GRAM suite provides a density variation of approximately 30% for the “relevant altitude ranges which is considered an optimistic estimate,” Girija writes. “Until in-situ data from an atmospheric probe becomes available, a more conservative global min-max estimate is recommended to accommodate the worst-case scenario.”
The altitude range of 200–400 km is the area where aerocapture would be most effective and Girija says the expected density variation of 30% “must be taken as an ‘optimistic’ estimate until in-situ data becomes available. The actual uncertainty may be much higher.”
Girija has written another paper, also posted to the arXiv preprint server, comparing lift and drag modulation for ice giant missions.
Overall, Girija says, the aerocapture mission design “must account for the expected atmospheric uncertainties to assure the guidance scheme can successfully steer the vehicle to the desired” location in the atmosphere or a landing. One of the most important parts of the mission design is the selection of the target entry flight path angle.
More information:
Athul Pradeepkumar Girija, Comparative Study of Planetary Atmospheric Uncertainties and Design Rules for Aerocapture Missions, arXiv (2023). DOI: 10.48550/arxiv.2310.10067
Athul Pradeepkumar Girija, Comparison of Lift and Drag Modulation Control for Ice Giant Aerocapture Missions, arXiv (2023). DOI: 10.48550/arxiv.2309.13812
Journal information:
arXiv
Provided by
Universe Today
Citation:
Aerocapture is a ‘free lunch’ in space exploration (2023, November 28)
retrieved 28 November 2023
from
This document is subject to copyright. Apart from any fair dealing for the purpose of private study or research, no
part may be reproduced without the written permission. The content is provided for information purposes only.