One of the chief objectives of the James Webb Space Telescope (JWST) is to study the formation and evolution of the earliest galaxies in the Universe, which emerged more than 13 billion years ago. To this end, scientists must identify galaxies from different cosmological epochs to explore how their properties have changed over time. This, in turn, requires precise dating techniques so astronomers are able to determine when (in the history of the Universe) an observed galaxy existed. The key is to measure the object’s redshift, which indicates how long its light has been traveling through space.
This is the purpose of the Cosmic Evolution Early Release Science Survey (CEERS), a collaborative research group that analyzes Webb data to learn more about galactic evolution. These galaxies are known as “high-redshift,” meaning that their light emissions are redshifted all the way into the infrared spectrum. Galaxies that existed ca. 13 billion years ago can only be observed in the near-infrared spectrum, which is now possible thanks to Webb’s Near-Infrared Camera (NIRCam). Even so, obtaining accurate redshift measurements from such distant galaxies is a very tricky, and requires advanced techniques.
When observing distant galaxies, astronomers will analyze their light using a spectrometer – a device that breaks light down into its respective wavelengths – to measure for redshift. In essence, redshift determines how long light has been traveling through space to reach us. Consistent with Einstein’s Special Theory of Relativity, we know that the speed of light is constant light’s regardless of the motion of the observer or source. However, since the space between the source and observer is constantly expanding, the light’s wavelength is elongated, causing it to shift towards the red end of the spectrum (hence the term “redshift”).
By obtaining accurate measurements of a galaxy’s redshift, astronomers can place it in the context of cosmic history. If a galaxy has a redshirt value of 6 (z = 6), astronomers will conclude that its light has been traveling through space for roughly 12.7 billion years. This means that the galaxy they are observing appears as it would have almost 13 billion years ago. Obtaining these values is not easy, but astronomers have come to rely on various techniques to make it easier. Micaela Bagley, a postdoctoral fellow at the University of Texas at Austin, is a member of the CEERS research group and is responsible for processing all the images taken by the Near-Infrared Camera (NIRCam).
As he explained in a recent NASA interview, two techniques involve measuring emission lines and using a “break” in a galactic spectrum – abrupt changes in the light intensity at specific wavelengths. A common break astronomers rely on is the spectral break of neutral hydrogen (or the Lyman Break), which corresponds to the amount of energy it can absorb before it becomes ionized. Similarly, there’s the Balmer break, where electrons become completely ionized directly from the second energy level of a hydrogen atom. Galaxies that show both of these breaks are called double-break galaxies.
Since astronomers know the wavelength of these breaks, they can target galaxies at certain distances by looking for breaks with the right redshift. Some of the first images Webb took were of double-break high redshift values (z = 7), meaning they existed when the Universe was less than one billion years old (ca. 13 billion years ago). However, these galaxies were much brighter and larger than expected, which did not sit well with prevailing cosmological models. This illustrates a flaw in the double-break method, which is great for finding galaxies but can introduce biases into the data. But as Bagley said, this is where the NIRCam instrument comes in handy:
“We take images using multiple filters to collect the object’s light in several different colors. When we measure a galaxy’s photometry, or how bright it is in an image, we’re measuring the brightness of the object averaged across the full range of wavelengths transmitted by the filter. We can observe a galaxy with NIRCam’s broadband imaging filters, but there is a lot of detailed information hidden within each single measurement for every 0.3–1.0 microns in wavelength coverage. Yet we can start to constrain the shape of a galaxy’s spectrum.”
The shape of a galaxy’s spectrum is affected by several properties, including the numbers of stars forming within it, how dusty it is, and how much its light has been redshifted. The measured brightness of the galaxy is then compared in each filter to what galaxy models predict, spanning a range of properties at a range of redshifts. Based on how well the model fits the data, astronomers can determine the galaxy is at a certain “moment in history.” This analytical process, where a “best fit” redshift is found, is known as the photometric redshift.
In July 2022, teams with the CEERS Survey used NIRCam images to identify two previously undetected galaxies with photometric redshifts greater than z = 11, corresponding to more than 13.4 billion years ago. Unfortunately, photometric redshift measurements of galaxies are also subject to a degree of uncertainty. As Bagley went on to explain, astronomers may be able to detect a spectral break in a filter but not the precise wavelength of the break:
“While we can estimate a best-fit redshift based on modeling the photometry, the resulting probability distribution is often broad. Additionally, galaxies at different redshifts can have similar colors in broadband filters, making it difficult to distinguish their redshifts based only on photometry. For example, red, dusty galaxies at redshifts less than 5 (or when the universe was 1.1 billion years old or older) and cool stars in our own galaxy can sometimes mimic the same colors of a high-redshift galaxy. We therefore consider all galaxies that are selected based on their photometric redshifts to be high-redshift candidates until we can obtain a more precise redshift.
To address this, astronomers will attempt to constrain a galaxy’s redshift by obtaining a spectrum, which is where Webb‘s Near-Infrared Spectrograph (NIRSpec) comes in. Astronomers can improve their calculations of redshift probability distribution by measuring the perceived brightness (photometry) of a galaxy in ever finer wavelengths. As they go from broadband filters to narrower filters to a spectrum, the distribution narrows until a very precise redshift measurement is obtained, known as a spectroscopic redshift.
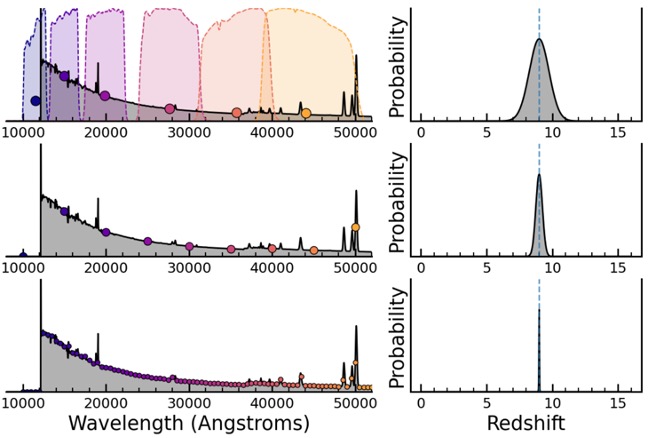
In February 2023, the CEERS team used the NIRSpec to obtain precise spectroscopic redshifts on two previously identified high-redshift candidates. One was Maisie’s Galaxy, which had a confirmed redshift of z = 11.4, corresponding to a distance of 13.4 billion light-years (when the Universe was 390 million years old). But when another team of astronomers analyzed the redshift measurements obtained by Webb, they determined that Maisie’s Galaxy had a redshift of z = 4.9 – corresponding to a distance of just over 12 billion light-years (about 1.2 billion years after the Big Bang).
“Even cases where we discover that a high-redshift candidate is actually a lower-redshift galaxy can be very exciting,” said Bagley. “They allow us to learn more about conditions in galaxies and the way those conditions affect their photometry, to improve our mod.els of galaxy spectra, and to constrain galaxy evolution across all redshifts. However, they also highlight the need to obtain spectra to confirm high-redshift candidates.”
Clearly, observing objects at cosmological distances and constraining their properties is no easy task. But with improved instruments and the sophisticated methods they allow, our measurements of the cosmos are rapidly improving. This, in turn, will allow astronomers to resolving the greatest cosmological mysteries of our time. These include the existence of Dark Matter and Dark Energy and the discrepancy between distance measurements to determine the rate of cosmic expansion – aka. the Hubble Tension. Slowly, but surely, we are getting closer to understanding how everything in our Universe fits together!
Further Reading: NASA